The Integral Nature of Encounter Rate in Predicting Livestock Depredation Risk
- 1Department of Fisheries and Wildlife, Michigan State University, East Lansing, MI, United States
- 2Conservation Science and Wildlife Health, San Diego Zoo Wildlife Alliance, Beckman Center for Conservation Research, Escondido, CA, United States
- 3Loisaba Conservancy, Laikipia, Kenya
- 4Wildlife Conservation Research Unit, Department of Zoology, The Recanati-Kaplan Centre, University of Oxford, Tubney, United Kingdom
Carnivore depredation of livestock is one of the primary drivers of human-carnivore conflict globally, threatening the well-being of livestock owners, and fueling large carnivore population declines. Interventions designed to reduce carnivore depredation typically center around predictions of depredation risk. However, these spatial risk models tend to be informed by data depicting the number of livestock attacked by carnivores. Importantly, such models omit key stages in the predation sequence which are required to predict predation risk, or in this case depredation risk. Applying the classic predation risk model defined by Lima and Dill demonstrates that depredation risk is dependent upon quantifying the rates at which carnivores encounter livestock before attacking. However, encounter rate is challenging to estimate, necessitating novel data collection systems. We developed and applied such a system to quantify carnivore-livestock encounters at livestock corrals (i.e., bomas) across a 9-month period in Central Kenya. Concurrently, we monitored the number of livestock attacked by carnivores at these bomas. We calculated carnivore-livestock encounter rates, attack rates, and depredation risk at the boma. We detected 1,383 instances in which carnivores encountered livestock at the bomas. However, we only recorded seven attacks. We found that the encounter rate and attack rate for spotted hyenas were almost six and three times higher than that for any other species, respectively. Consequently, spotted hyenas posed the greatest depredation risk for livestock at the boma. We argue that better understanding of carnivore-livestock encounter rates is necessary for effective prediction and mitigation of carnivore depredation of livestock.
Introduction
Biodiversity loss is a wicked problem yielding a number of negative impacts on coupled human and natural systems around the world (Chapin et al., 2000; Pimm et al., 2014; Beck et al., 2019). In the dynamic and increasingly-globalized twenty-first century, drivers of biodiversity loss are varied, ranging from coarse processes including climate change and land conversion, to fine-scale ones such as poaching (Chapin et al., 2000; Pimm et al., 2014). Loss of biodiversity in the order Carnivora is of particular concern given the influential role that these species tend to play in the apex and meso-predator positions of global ecosystems (Estes et al., 2011; Ripple et al., 2014; Dorresteijn et al., 2015). As these roles are often near the top of trophic systems, large carnivores can be key ecological regulators facilitating the maintenance of ecosystem health (Estes et al., 2011; Ripple et al., 2014). Despite their ecological importance, >75% of the world's remaining large carnivore populations have declining trajectories (Ripple et al., 2014, 2016; Wolf and Ripple, 2017) principally driven by habitat loss and fragmentation, overhunting, prey depletion, and conflict with humans (Dickman, 2010; Ripple et al., 2014; Eklund et al., 2017; Wolf and Ripple, 2017; Krafte Holland et al., 2018). Conflict between humans and carnivores tends to be associated with threats to human security and private property (Ripple et al., 2014; van Eeden et al., 2018a,b). Most common among these threats is depredation of livestock, where retaliatory killing of carnivores by affected livestock owners is a common response (Krafte Holland et al., 2018; van Eeden et al., 2018b). Consequently, carnivore depredation of livestock is an important challenge facing both biodiversity conservation and human wellbeing (Barua et al., 2013).
Carnivore depredation of livestock persists globally and has expanded with increasing human population growth (Wolf and Ripple, 2017). To date, there is minimal evidence of the effectiveness of most applied solutions to this conflict (Miller, 2015; Eklund et al., 2017; van Eeden et al., 2018a). This limited efficacy is attributable, in part, to evident disconnects between research effort and the implementation of conservation strategies (Sunderland et al., 2009; Montgomery et al., 2018a; Beck et al., 2019; Gray et al., 2019). One potential driver of this research-implementation gap involves the misalignment of research practice and conservation need (Linklater, 2003; Lawler et al., 2006; Stroud et al., 2014; Di Marco et al., 2017). This facet of the research-implementation gap is influenced by a range of factors including positive feedbacks in patterns of conservation funding (Stroud et al., 2014; Troudet et al., 2017) and taxonomic biases in publication review and editing decisions (Bonnet et al., 2002; Linklater, 2003; Martín-López et al., 2009). Further, research on carnivore depredation of livestock is often restricted to well-established methodologies such as fecal analysis and track transects because of the logistical constraints associated with remote study locations and dynamic animal behaviors (Van der Weyde et al., 2018; Prugh et al., 2019). Consequently, in many cases research practices have been slow in adapting to meet changing conservation needs (Lawler et al., 2006; Stroud et al., 2014). As a result, critical subjects remain unexplored in the human-carnivore conflict literature, one of which is the rate at which carnivores encounter potential livestock prey.
Predator-prey encounters are a fundamental stage of the predation sequence (Mech, 1970; Lima and Dill, 1990; MacNulty et al., 2007) and encounter rate is an integral parameter necessary to predict predation risk (see Holling, 1959; Lima and Dill, 1990; Abrams, 2000). In the predator-prey model originally presented by Holling (1959) and adapted by Lima and Dill (1990), predation risk can be predicted as a function of:
Where the probability of prey being killed P(death) is derived from the rate of encounter between predator and prey (α), the probability of death given an encounter (d), and the time spent vulnerable to an encounter (T; Lima and Dill, 1990). The probability of death given an encounter (d) is calculated by the conditional probabilities (i.e., “subcomponents” of predation risk) of various possible interactions between predator and prey (Figure 1). Specifically, d is defined as:
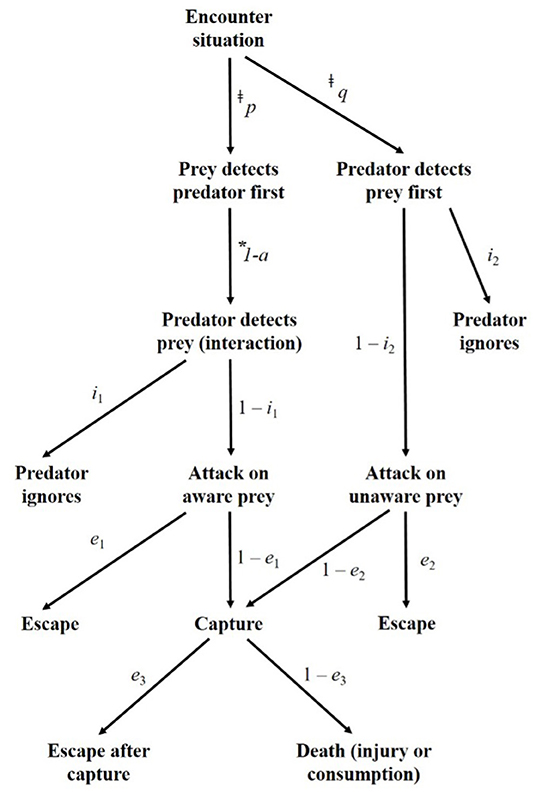
Figure 1. Flow chart of the potential outcomes of carnivore-livestock encounters at the boma, adapted from Lima and Dill (1990). The text next to the arrows indicate the conditional probabilities associated with each pathway. ‡We defined all instances in which carnivores were detected on a camera to be encounters, thus p + q = 1. *Bomas restrict livestock movement, removing the possibility of avoidance prior to detection by a carnivore. Therefore, 1 – a = 1.
Here, a represents the probability of effective avoidance of the predator, which is not a possible outcome in the case of corralled livestock, i1 and i2 are attack subcomponents, and e1, 2, and3 are escape subcomponents. Importantly, this model predicting predation risk cannot be fit without an understanding of the rates at which predators encounter prey (Holling, 1959; Curio, 1976; Lima and Dill, 1990; Abrams, 2000; Prugh et al., 2019). Despite the importance of encounter rate, depredation risk is typically calculated without this integral parameter.
Depredation risk models are most often informed solely by successful carnivore attack on livestock data (Treves et al., 2004; Miller, 2015; Hoffmann et al., 2019). These models, often termed “spatial risk models,” typically use the count of these attacks to associate abiotic and biotic conditions with depredation (Hebblewhite et al., 2005; Baruch-Mordo et al., 2008; Behdarvand et al., 2014; Miller et al., 2015; Broekhuis et al., 2017). Here, carnivore depredation data are collated across space and time to map spatial, and sometimes temporal, variation in depredation risk. The accuracy of these models is paramount given that the spatio-temporal predictions regularly inform interventions designed to reduce human-carnivore conflict (Miller, 2015; Mpakairi et al., 2018; Hoffmann et al., 2019). As described by Miller (2015), “spatial risk models quantify the realized predation risk…rather than the overall fundamental predation risk.” Therefore, these models do not quantify depredation risk (sensu the predation risk equation of Lima and Dill, 1990), but instead use only the final step in the predation sequence of potential interactions and outcomes (Figure 1) as a proxy for depredation risk. Hereafter, we refer to depredation risk as defined by Lima and Dill (1990), not as this realized predation proxy. Notably, while studies relying on this proxy provide valuable insights that can inform livestock husbandry and carnivore management strategies, they may be limited in their ability to predict predation risk as they omit measures of encounter rate.
Carnivore-livestock encounters have likely not been widely estimated because of the challenges inherent to collecting these types of data. Efforts to collect encounter data are often hampered by the cryptic and wide-ranging nature of carnivores as well as the technological limitations in mapping contact points between carnivores and livestock (Lima, 2002; Breck, 2004; Gray, 2012; Krafte Holland et al., 2018). Consequently, novel means of data collection are required to overcome these constraints. We developed and deployed such a system to detect carnivore-livestock encounters. We applied this system to a region of Central Kenya that experiences high rates of carnivore depredation of livestock (Ogada et al., 2003; Frank, 2010). Concurrently, we also collected data on carnivore attacks on livestock, in the same manner as is traditionally used in the aforementioned spatial risk modeling. We estimated species-specific carnivore encounter rates, attack rates, and calculated depredation risk using the Lima and Dill (1990) equation. As rates of livestock depredation, and the resultant impacts on both humans and carnivores, are likely to continue to intensify, targeted examinations of the applicability of research practice to conservation needs are increasingly necessary. Our contribution to this effort here provides valuable insights into carnivore space use and habitat selection, mitigation efforts informed by those behaviors, and recommendations for future studies to better elucidate the stochasticity that remains unexplored in patterns of carnivore depredation of livestock.
Methods
Study Area
We positioned our study in Central Kenya, along the borders of Samburu, Isiolo, and Laikipia Counties (Figure 1). This landscape is predominantly semi-arid bushland divided among a matrix of privately-owned conservancies, commercial ranches, and agro-pastoralist community-owned conservancies (Frank et al., 2005; Frank, 2010; Bond, 2014; Yurco, 2017). Livestock-owners in this landscape keep cattle, sheep, and goats (collectively referred to as shoats), donkeys, and camels (Ogada et al., 2003; Frank et al., 2005; Frank, 2010; Unks et al., 2019). There are two rainfall seasons, with heavier rains from April-June and lighter rains from October-December. Total annual rainfall is highly variable, but often ranges from 500 to 750 mm (Mizutani, 1999a; Georgiadis et al., 2007). The landscape supports a carnivore guild of African lions (Panthera leo), leopards (Panthera pardus), cheetahs (Acinonyx jubatus), spotted hyenas (Crocuta crocuta), striped hyenas (Hyaena hyaena), black-backed jackals (Canis mesomelas), aardwolves (Proteles cristata), caracals (Caracal caracal), and African wild dogs (Lycaon pictus; Mizutani, 1999b; Ogada et al., 2003; Frank et al., 2005). All of these carnivore species, with the exception of aardwolves have been found to depredate livestock in this region (Frank et al., 2005; Frank, 2010).
Our 650 km2 study area included the western units of Oldonyiro Community Conservancy, the southern units of Kirimon Community Conservancy, and the Koija and Il Motiok units within Naibunga Community Conservancy, which are subject to considerable levels of carnivore depredation of livestock (Figure 2). For instance, between March, 2018 and October, 2020; livestock-owners in these communities reported 2,390 livestock injured or killed by large carnivores, 67% of which were attacked at night while held in livestock corrals within homesteads, otherwise known as bomas (Pilfold and Ruppert, unpublished data). Therefore, we focused our data collection system on detecting carnivores encountering and attacking livestock at the boma.
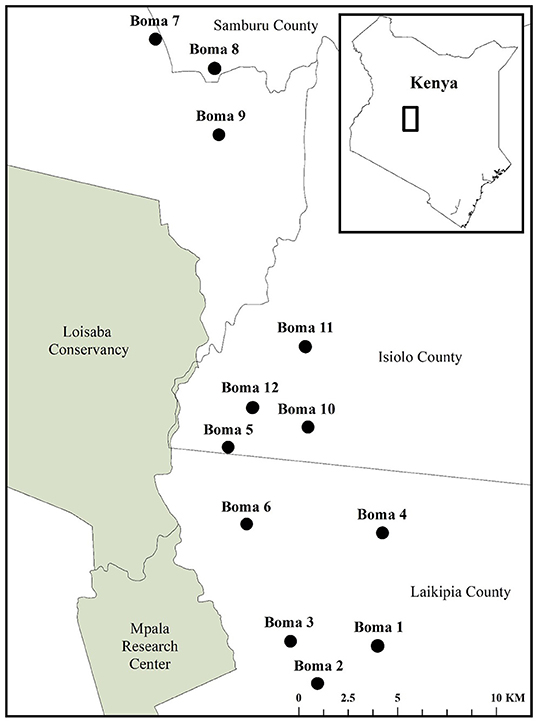
Figure 2. The location of 12 bomas equipped with the motion-activated cameras in Central Kenya to detect carnivore species encountering livestock from September, 2018 to May, 2019.
Data Collection
We selected 12 bomas across our study site via a stratified random sample, with three bomas randomly chosen within each of the four communities (Figure 2). Upon identifying these bomas, we consulted the chairman of each community and met with the heads of all households to gain permission for their participation in our study. We equipped each boma with a suite of motion-activated cameras (Bushnell Trophy Cam HD Aggressor—No Glow, Model 119776C) attached to fence posts, and positioned them to be outward-facing. We placed all cameras 0.5 m off of the ground and evenly distributed around the outer wall of the boma so as to best detect approaching carnivores. Each boma had 6–7 cameras depending on the circumference of the perimeter wall, separated by an average distance of 27.1 m (Figure 3). We programmed the cameras to record videos (15-s duration) with no delay period between triggers. The cameras were active between dusk and dawn, consistent with the time period in which livestock are vulnerable to carnivore depredation at the boma (Ogada et al., 2003; Frank et al., 2005; Kissui, 2008). We maintained these cameras across the duration of our study, between September, 2018 and May, 2019.
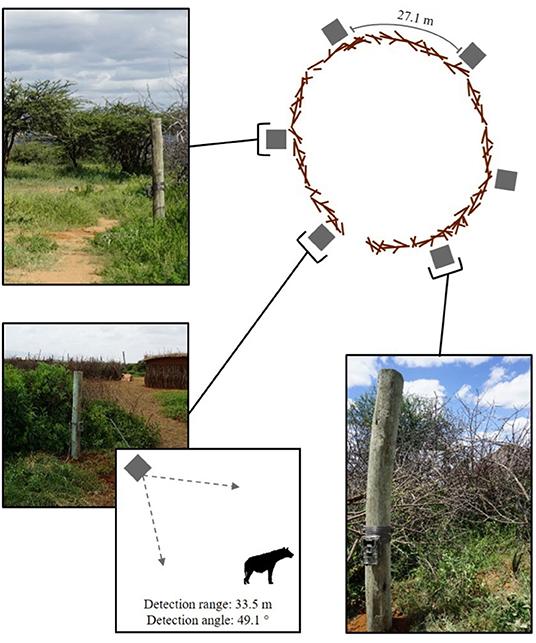
Figure 3. The location of the motion-activated cameras around the perimeter of one the 12 bomas in Central Kenya. The diagram indicates the position of the cameras in relation to the boma wall, the average distance between cameras, and the camera detection range and angle. The photographs show the placement of the cameras on the fence posts.
Over the same time period, we followed established protocols (see Ogada et al., 2003; Woodroffe et al., 2005; Kissui, 2008; Leflore et al., 2019) to map the frequency of carnivore attacks on livestock at these bomas. We documented attack records via a conflict reporting network composed of 19 community representatives selected by local leadership (see Ruppert et al., 2021). Livestock owners were asked to call their representative immediately following each conflict event involving carnivores. At the incident site, representatives used a structured questionnaire to gather data from the livestock owner and, when available, a witness. At each event, the representative recorded the; (i) date and approximate time of the attack, (ii) type and number of livestock injured or killed, (iii) carnivore species responsible, and (iv) GPS location of the attack. Identification of responsible carnivore species was determined as soon as a representative could reach the site, with species determined via tracks, bite location and marks, and any other evidence associated with the incident site, with consensus reached among representatives, livestock owners, and a researcher or witness when available. We also confirmed the presence of the responsible species at the attacked boma via camera trap videos for each incident during the study period. Representatives were initially trained to administer the standardized form in March 2018, and guidance on the process and use of equipment were reinforced during monthly field visits by AL, LL, and IL. All representatives entered the data directly into Survey 123 software on Samsung Galaxy J1 phones while at the location of the attack. Ethical approvals for human subjects research were reviewed and approved by the Institutional Review Board (IRB #02555e) of Miami University, Ohio. Permission was granted by the Kenya National Commission for Science, Technology, and Innovation under Research License #690384, and Kenya Wildlife Services. Methods were also reviewed and approved by the Institutional Animal Care and Use Committee at San Diego Zoo Global (Protocol #18-017).
Data Analysis
For each video file returned from the motion-activated cameras, we recorded the: (i) carnivore species detected, (ii) number of individuals of that species, (iii) date, and (iv) time the camera was triggered. We defined a carnivore-livestock encounter have occurred when at least one individual of a depredating carnivore species of was detected in the viewshed of the cameras (i.e., within 33.5 m of the boma wall, Figure 3). We considered instances when multiple individuals of the same species were present at the boma concurrently to be one encounter event. The detection extent is consistent with the chase initiation distance of the carnivore species (Kruuk, 1972; Elliot et al., 1977; Hayward et al., 2006) and thus, representative of an encounter (Lima and Dill, 1990). However, carnivore-livestock interactions are unique in comparison to free-ranging prey encounters due to the structural interference of the boma wall, which can influence attack dynamics. Regardless, the walls of the 12 bomas were all constructed with acacia thorn bush with highly variable structural integrity, often resulting in openings large enough to allow for visibility through the physical barrier (Lichtenfeld et al., 2015; Chaka et al., 2020). Further, carnivores regularly break into bomas to attack livestock, demonstrating they are aware of the presence of livestock within (Ogada et al., 2003; Kolowski and Holekamp, 2006; Kissui, 2008). Livestock also often stampede in an attempt to flee the boma when carnivores are near, indicating they are similarly aware of the presence of carnivores on the other side of the wall (Ogada et al., 2003; Frank, 2010). To limit pseudo replication, we consolidated all videos of one carnivore species triggered within 10 min of each other at the same boma into a single encounter. We constrained our encounters to a 10 min window, as previous examinations indicate that species detections decrease significantly outside of this time frame (Lepard et al., 2019).
We quantified the number of encounters and attacks per night, and examined variation in carnivore-livestock encounters by date, time, boma location, and carnivore species. We then calculated the species-specific depredation risk for livestock in the boma using Equations (1, 2). We based these calculations upon the rate of encounter, and the number of attacks by each carnivore species resulting in livestock escape (i.e., no injury), escape after capture (i.e., injury), and death. As noted above, we defined all instances in which a carnivore was detected on a camera to be an encounter. Consequently, we assumed p + q = 1, as all encounters progressed to the next stage of the predation sequence (see Lima and Dill, 1990; Figure 1). Similarly, we assumed 1 – a = 1 because bomas constrain the movement of livestock (Frank et al., 2006; Frank, 2010; Chaka et al., 2020). When encounters occur with livestock corralled within a boma, they do not have the freedom of movement to avoid carnivores prior to detection. By the same logic, whether the livestock or carnivore detect the other first is likely to have little effect on subsequent progression through the stages of predation. Therefore, we made the following further assumptions: (i) depredation risk was equal for both orders of detection (p = q), (ii) risk of attack was equal regardless of whether or not the livestock are aware of carnivore presence (1 – i1 = 1 – i2), and (iii) risk of capture was equal regardless of whether or not the livestock were aware of carnivore presence (1 – e1 = 1 – e2; Figure 1).
Results
Between September, 2018 and May, 2019 our motion-activated cameras recorded a total of 2,347 videos of carnivores detected at 12 livestock bomas, of which there were a total of 1,383 independent carnivore-livestock encounters. The five species identified among these carnivore-livestock encounters were spotted hyenas, striped hyenas, black-backed jackals, leopards, and African lions (Figures 4, 5A, 6). The most common carnivore species that we detected was the spotted hyena, which encountered livestock at the bomas in 76.1% (n = 1,052) of the detections. The next most detected species was the striped hyena (13.2%, n = 183), followed by black-backed jackals (10.1%, n = 139), leopards (0.5%, n = 7), and African lions (0.1%, n = 1; Table 1; Figures 5A, 6). The number of carnivore-livestock encounters increased throughout the evening hours, peaking between 23:00 and 03:00 before declining to 06:00 (Figure 5B). Carnivore-livestock encounters also fluctuated throughout the study period, with peaks in November, 2018 and May, 2019 corresponding to the light and heavy rainy seasons, respectively (Figure 5C). The number of carnivore-livestock encounters also varied by boma, ranging from 26 to 472 (Figures 5D, 6). Across the same time period, we recorded a total of seven carnivore attacks on livestock at the study bomas. Only two carnivores, spotted hyenas (71.4%, n = 5) and leopards (28.6%, n = 2), were responsible for these livestock attacks (Tables 1, 2). The attacks resulted in the deaths of one dog and 21 shoats, and the injury of two additional shoats (Table 2).
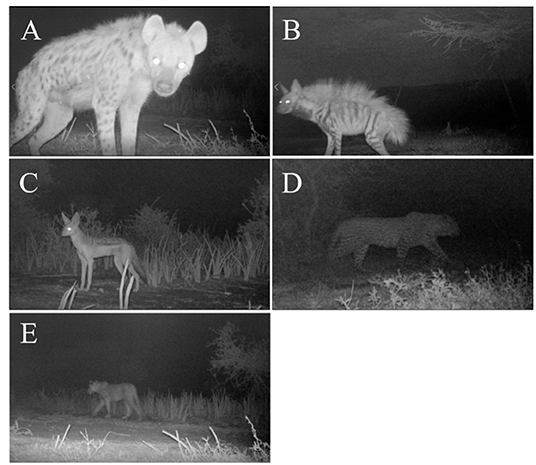
Figure 4. The carnivore species detected on motion-activated cameras at 12 bomas in Central Kenya from September, 2018 to May, 2019. The five potentially depredating species recorded to encounter livestock were: (A) Spotted hyena (Crocuta crocuta), (B) Striped hyena (Hyaena hyaena), (C) Black-backed jackal (Canis mesomelas), (D) Leopard (Panthera pardus), and (E) African lion (Panthera leo).
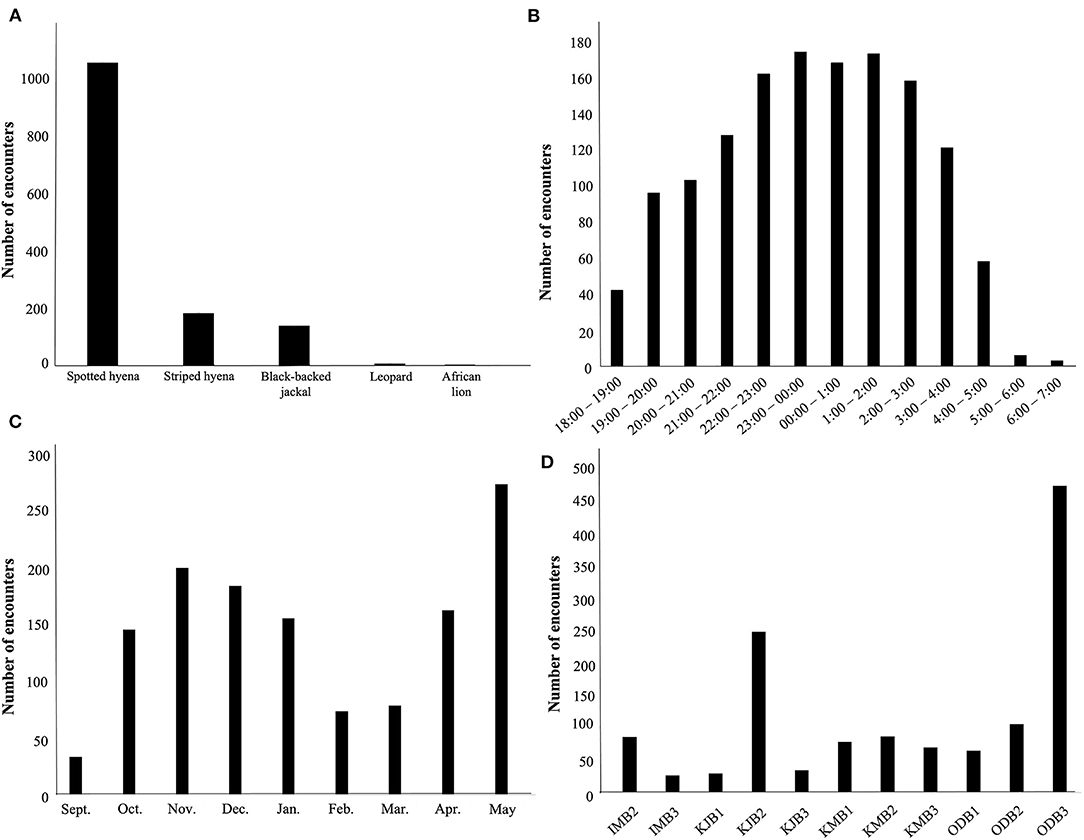
Figure 5. The patterns of carnivore-livestock encounters at 12 bomas in Central Kenya from September, 2018 to May, 2019. The panels show the number of carnivore-livestock encounters by: (A) species, (B) time, (C) month, and (D) boma.
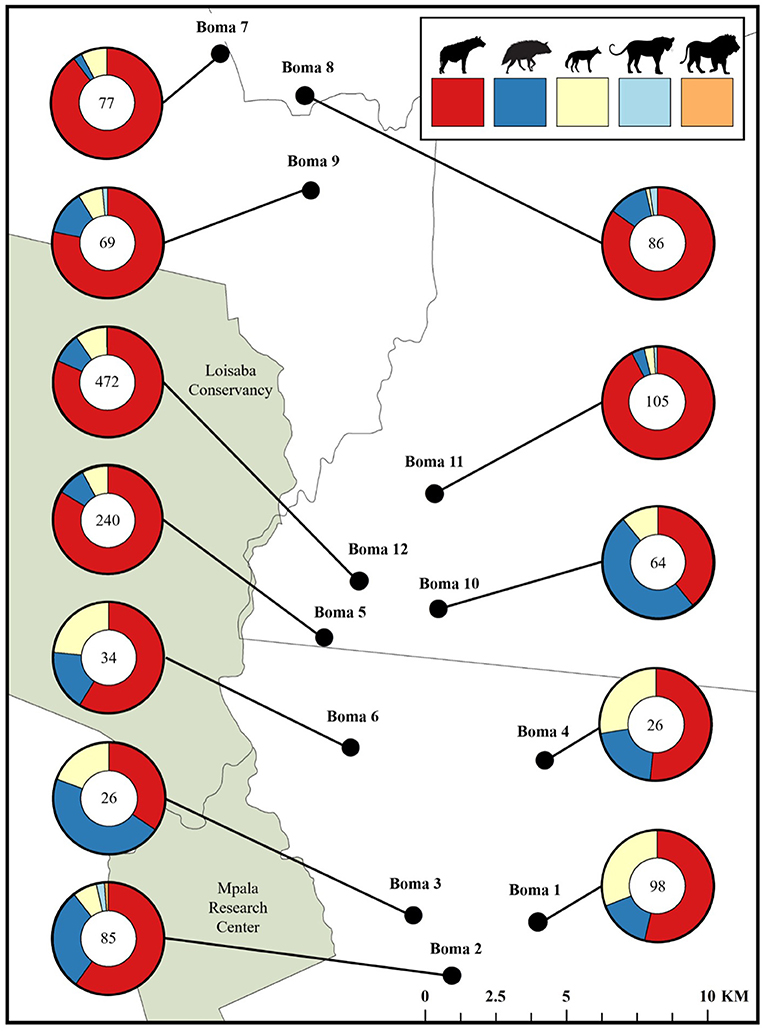
Figure 6. The proportion of carnivore-livestock encounters attributed to each of the five recorded carnivore species at 12 bomas in Central Kenya from September, 2018 to May, 2019. Each chart also indicates the total number of carnivore-livestock encounters at the boma.
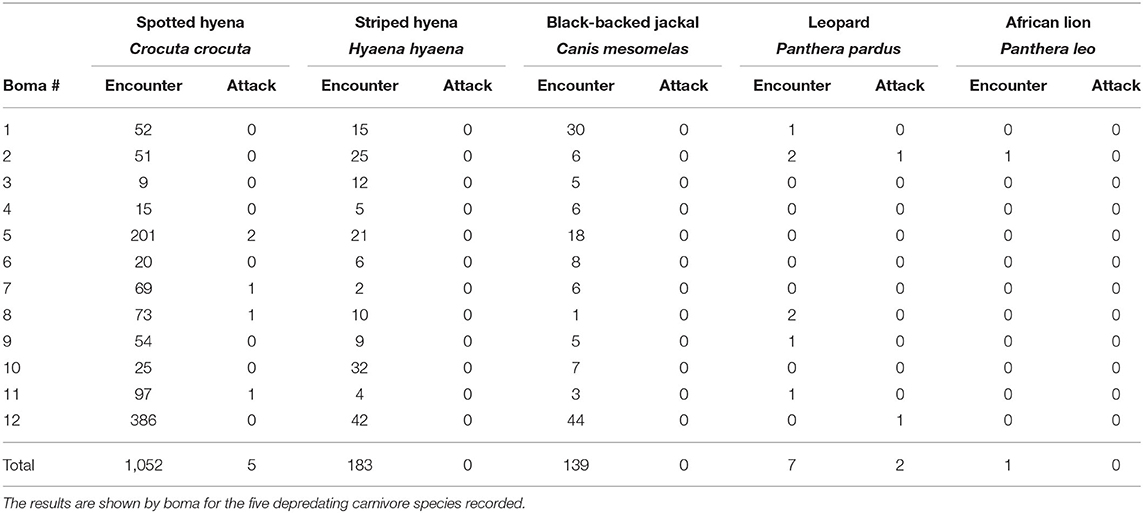
Table 1. The number of carnivore-livestock encounters and carnivore attacks on livestock at 12 bomas in Central Kenya from September, 2018 to May, 2019.
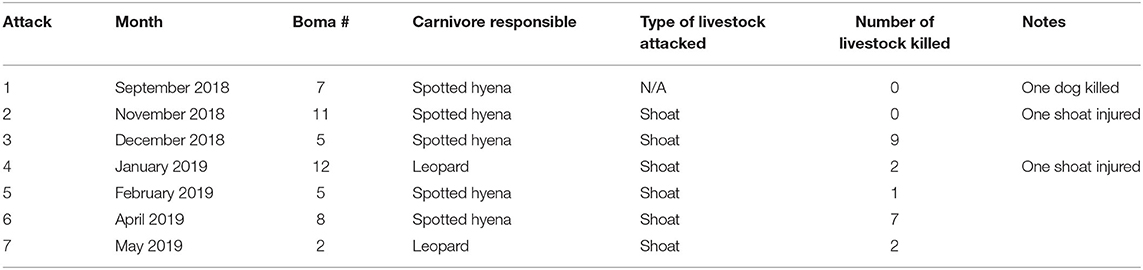
Table 2. The seven carnivore attacks on livestock reported at 12 bomas in Central Kenya from September, 2018 to May, 2019.
We recorded the highest encounter rate for spotted hyenas, with an average of 3.79 encounters/night (Table 3). Striped hyenas had the next highest rate (0.66 encounters/night), followed by black-backed jackals (0.51 encounters/night), leopards (0.03 encounters/night), and African lions (0.004 encounters/night; Table 3). Spotted hyenas and leopards were the only species with non-zero attack rates and calculated depredation risk. Spotted hyenas averaged 0.02 attacks/night, while leopards averaged 0.007 attacks/night. Depredation risk from spotted hyenas per boma was 0.01, and 0.007 for leopards (Table 3).
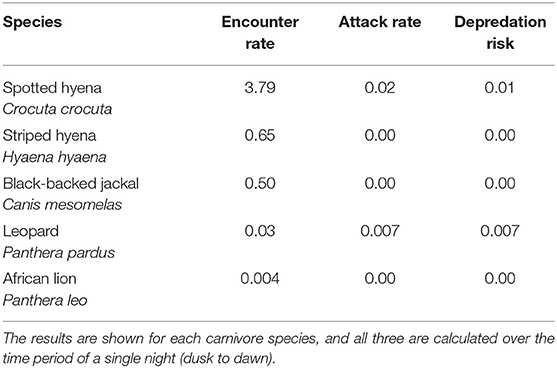
Table 3. The average encounter rate, attack rate, and depredation risk at 12 bomas in Central Kenya from September, 2018 to May, 2019.
Discussion
Assessments of the research-implementation gap regularly identify factors that diminish the impact of applied conservation practice (Knight et al., 2008; Miller et al., 2016). Within the context of human-carnivore conflict, effective alignment between data collection and conservation need is of particular importance (Montgomery et al., 2018a; Beck et al., 2019; Gray et al., 2019; Hoffmann and Montgomery, 2022). Our study supports this broader research effort by demonstrating the integral nature of encounters in predicting carnivore depredation of livestock risk. We found that spotted hyenas had the highest encounter rate among all carnivores identified, with the average number of encounters per night almost six times higher than any other species. However, the depredation risk associated with spotted hyenas was only marginally higher than that of leopards, which were the next most common depredator. Therefore, our results indicate that carnivore species exhibit vastly different depredatory behaviors at the boma. Furthermore, we found that carnivores encountered livestock at the boma far more often than they attacked (Tables 1, 3).
While the number of carnivore attacks of livestock was low overall, we recorded carnivore-livestock encounters at all study bomas, with some experiencing hundreds of encounters during the study period (Figures 5D, 6). However, the fact that the direct effects of carnivore depredation were low does not mean that the indirect effects of carnivore presence were inconsequential. Carnivore presence may have substantial effects on both the livestock and the overall conflict driven by livestock depredation. For instance, carnivore presence influences the behavior of cattle in Eastern African grazing landscapes, which may result in reduced foraging (Beck et al., 2020). Impacts such as these, often termed non-consumptive effects or risk effects, have been well-studied in both wild prey systems and grazing livestock herds (Fortin et al., 2004; Basille et al., 2015; Moll et al., 2017; Beck et al., 2020). Additionally, interactions with predators may increase livestock stress levels and modify vigilance behavior, resulting in a range of impacts including reduction of body condition and decreased reproductive output (Lima, 1998; Creel et al., 2007; Creel and Christianson, 2008; Laporte et al., 2010). While we are not aware of any study that has examined these types of impacts at the scale of bomas (Montgomery et al., 2018b), it is likely that carnivore presence is similarly impacting the livestock corralled within these structures. As our study presents a limited sample of 12 bomas across 1 year, we offer the non-consumptive effects of carnivore-livestock encounters at the boma as a rich area of future inquiry.
We also detected substantial differences in encounter rates among the carnivore species recorded. Spotted hyenas accounted for over three quarters (76.1%) of recorded encounters (Table 1; Figures 5A, 6). Similarly, they were responsible for 71.4% of attacks on livestock, resulting in the injury or death of 18 shoats, and had the highest calculated depredation risk (Tables 1–3). This finding aligns with those in other examinations of livestock depredation in both Laikipia County and sub-Saharan Africa more broadly, in which spotted hyenas are commonly reported to be the primary cause of livestock depredation (Ogada et al., 2003; Kissui, 2008; Frank, 2010; Mponzi et al., 2014; Kissui et al., 2019; Hoffmann and Montgomery, 2022). Relative carnivore population densities often provide a logical explanation for species-specific variation in livestock depredation (Kolowski and Holekamp, 2006). However, best estimates indicate that there is unlikely to be a significant difference in population density among the species we recorded encountering livestock at the boma (Frank, 1998; Frank et al., 2005; Wagner, 2006; Kinnaird and O'Brien, 2012; Prager et al., 2012; Bauer et al., 2016). Thus, the variation we identified among the rates of attack and encounter by carnivore species may be due to alternative drivers.
One potential explanation for this species-specific variation comes from the foraging and movement behavior of each species. We found that leopards attacked livestock once out of every four encounters at the boma, whereas spotted hyenas attacked only once out of every 211 encounters. Additionally, while we only recorded two leopard attacks, both resulted in the death of livestock. In contrast, livestock were killed in only slightly over half of the recorded spotted hyena attacks. Importantly, our small sample size precludes us from drawing any concrete conclusions related to these depredatory behaviors. However, our results provide a preliminary indication that the risk of death, given encounter (d in Equation 1), may vary substantially by carnivore species. Spotted hyenas are widely recognized as opportunistic feeders and are commonly recorded scavenging from human landscapes (Kolowski and Holekamp, 2006, 2007; Abay et al., 2011). They may approach bomas in search of refuse, subsequently attacking nearby livestock as those opportunities arise (Kolowski and Holekamp, 2007; Yirga et al., 2015; Chaka et al., 2020). It is therefore likely that spotted hyena-livestock encounters are driven not only by depredatory behaviors, but also by scavenging opportunities (Yirga et al., 2014). As spotted hyenas were the most common depredator of livestock in our study and many others, those scavenging opportunities are likely a key contributing factor to the depredation risk associated with that species. Consequently, the effectiveness of livestock depredation interventions for spotted hyenas may be improved via increased emphasis on reducing encounter rates. For example, efforts to minimize scavenging attractants for spotted hyenas at the boma (e.g., secure off-site butchering locations, better waste management infrastructure) may reduce encounter rates and thus depredation risk (Chaka et al., 2020).
Examination of temporal variation in carnivore-livestock encounters also revealed patterns relevant to the mitigation of depredation risk. We identified a peak in the frequency of carnivore-livestock encounters between the hours of 23:00 and 03:00, with a steep drop-off in the frequency of encounters in the early morning hours (Figure 5B). This result corresponds to trends in carnivore behaviors identified in other studies. For example, Cozzi et al. (2012) recorded a similar reduction in spotted hyena movement between midnight and sunrise. They identified nighttime light availability as the driving force of this trend, with the carnivores maximizing their activity during the darkest part of the night. The potentially depredating carnivores recorded in our encounters also are primarily nocturnal hunters (Hopcraft et al., 2005; Hayward et al., 2006; Van Cleave et al., 2018), and therefore are likely to exhibit similar activity patterns. Depredation risk is generally considered to be high between dusk and dawn, as most livestock are killed at night (Ogada et al., 2003; Yirga et al., 2012). The temporal variation we identified in carnivore-livestock encounters, however, shows that there may be fluctuations in depredation risk across that high-risk time period. The conditions associated with when a carnivore chooses to approach the boma may also correlate with prevailing biotic and abiotic conditions, including stochasticity in human behavior. Few households in this region have access to consistent electrical power, so human activity tends to closely align with natural light availability. Consequently, factors that have been shown to act as carnivore deterrents, such as human voices and dog vigilance (Ogada et al., 2003; Frank, 2010), are likely to be minimal between 23:00 and 03:00. However, the role of these fine-scale factors in deterring carnivore encounters, and subsequent attacks on livestock, requires further investigation.
We also identified variation in carnivore-livestock encounters by season. For instance, there was one peak in carnivore-livestock encounters in November and another in June (Figure 5C). These peaks closely correlate with the timing of the two wet seasons in our study area. Specifically, the highest numbers of carnivore-livestock encounters aligned with periods of greatest mean rainfall (Mizutani, 1999a; Ulrich et al., 2012). The influence of seasonality on carnivore depredation of livestock has been examined extensively across sub-Saharan Africa (Patterson et al., 2004; Woodroffe and Frank, 2005; Kolowski and Holekamp, 2006; Valeix et al., 2009; Mukeka et al., 2019; Robertson et al., 2019). Consistently, rates of carnivore depredation of livestock have been shown to increase during rainy seasons (but see Pozo et al., 2020). This seasonality has been attributed to many potential drivers, including herding practices, wild prey migration, and prey switching driven by reduced predation success on wild prey (Patterson et al., 2004; Kolowski and Holekamp, 2006; Valeix et al., 2012; Mponzi et al., 2014; Kuiper et al., 2015; Loveridge et al., 2017; Kissui et al., 2019; Mukeka et al., 2019). As far as we are aware, however, this study provides the first evidence of seasonal variation in the frequency of carnivore-livestock encounters. This distinction is important, as it indicates that the well-established seasonal trends in rates of carnivore depredation of livestock are not just associated with the probability of death, given an encounter (d; Equation 1) or increased time spent vulnerable to an encounter (T), but are in fact likely associated with an increase in the rate of encounter itself (α).
Conclusions
Prediction of depredation risk is a fundamental strategy among studies seeking to mitigate carnivore depredation of livestock. Yet, logistical and ecological constraints have largely narrowed such approaches to the application of a proxy that cannot capture all aspects of depredation risk. Our effort to address this limitation highlights the important role of encounters in this predator-prey system. Encounter rate is an essential component of the prediction of depredation risk, and the subsequent development of interventions to minimize carnivore depredation of livestock. The evident variation in carnivore-livestock encounter rates and depredation risk by species further indicates the value of carnivore-livestock encounters in informing depredation mitigation efforts. However, there are many avenues of research that remain to be fully explored in relation to carnivore-livestock encounters. While the methods presented herein are limited to corralled livestock, recent advancements in animal-borne technologies such as proximity collars and neck-mounted cameras provide opportunities to explore encounter rates among mobile prey as well (Wilmers et al., 2015; Prugh et al., 2019). We advocate for further examination of encounters in multiple carnivore-livestock scenarios to develop more effective livestock protection efforts and continue to narrow the research-implementation gap.
Data Availability Statement
The raw data supporting the conclusions of this article will be made available by the authors, without undue reservation.
Ethics Statement
The studies involving human participants were reviewed and approved by Institutional Review Board (IRB #02555e) of Miami University, Ohio. Written informed consent for participation was not required for this study in accordance with the national legislation and the institutional requirements. The animal study was reviewed and approved by Institutional Animal Care and Use Committee at San Diego Zoo Global (Protocol #18-017).
Author Contributions
CH, NP, KR, and RM conceived the ideas and designed methodology. CH, NP, KR, AL, LL, and IL collected the data. CH analyzed the data. CH and RM led the writing of the manuscript. All authors contributed critically to the study and gave final approval for publication.
Funding
CH was supported by the University Fellowship Program at Michigan State University, but this research received no specific grant from any funding agency, or commercial or not-for-profit sectors. Funding for open access publication fees is provided by the San Diego Zoo Wildlife Alliance.
Conflict of Interest
AL, LL, and IL were employed by Loisaba Conservancy.
The remaining authors declare that the research was conducted in the absence of any commercial or financial relationships that could be construed as a potential conflict of interest.
Publisher's Note
All claims expressed in this article are solely those of the authors and do not necessarily represent those of their affiliated organizations, or those of the publisher, the editors and the reviewers. Any product that may be evaluated in this article, or claim that may be made by its manufacturer, is not guaranteed or endorsed by the publisher.
References
Abay, G. Y., Bauer, H., Gebrihiwot, K., and Deckers, J. (2011). Peri-urban spotted hyena (Crocuta crocuta) in Northern Ethiopia: diet, economic impact, and abundance. Euro. J. Wildlife Res. 57, 759–765. doi: 10.1007/s10344-010-0484-8
Abrams, P. A.. (2000). The evolution of predator-prey interactions: theory and evidence. Ann. Rev. Ecol. Syst. 31, 79–105. doi: 10.1146/annurev.ecolsys.31.1.79
Barua, M., Bhagwat, S. A., and Jadhav, S. (2013). The hidden dimensions of human-wildlife conflict: health impacts, opportunity and transaction costs. Biol. Conserv. 157, 309–316. doi: 10.1016/j.biocon.2012.07.014
Baruch-Mordo, S., Breck, S. W., and Wilson, K. R. (2008). Spatiotemporal distribution of black bear-human conflicts in Colorado, USA. J. Wildlife Manag. 72, 1853–1862. doi: 10.2193/2007-442
Basille, M., Fortin, D., Dussault, C., Bastille-Rousseau, G., Ouellet, J. P., and Courtois, R. (2015). Plastic response of fearful prey to the spatiotemporal dynamics of predator distribution. Ecology 96, 2622–2631. doi: 10.1890/14-1706.1
Bauer, H., Packer, C., Funston, P. F., Henschel, P., and Nowell, K. (2016). Panthera leo (errata version published in 2017). The IUCN Red List of Threatened Species 2016: e.T15951A115130419. doi: 10.2305/IUCN.UK.2016-3.RLTS.T15951A107265605.en
Beck, J. M., Lopez, M. C., Mudumba, T., and Montgomery, R. A. (2019). Improving human-lion conflict research through interdisciplinarity. Front. Ecol. Evol. 7, 1–8. doi: 10.3389/fevo.2019.00243
Beck, J. M., Moll, R. J., Kissui, B. M., and Montgomery, R. A. (2020). Do Pastoralist cattle fear African lions? Oikos. 130, 422–430. doi: 10.1111/oik.07965
Behdarvand, N., Kaboli, M., Ahmadi, M., Nourani, E., Mahini, S., and Aghbolaghi, A. (2014). Spatial risk model and mitigation implications for wolf–human conflict in a highly modified agroecosystem in western Iran. Biol. Conserv. 177, 156–164. doi: 10.1016/j.biocon.2014.06.024
Bond, J.. (2014). Conflict, development and security at the agro–pastoral–wildlife nexus: a case of Laikipia County, Kenya. J. Dev. Stud. 50, 991–1008. doi: 10.1080/00220388.2014.909025
Bonnet, X., Shine, R., and Lourdais, O. (2002). Taxonomic chauvinism. TRENDS Ecol. Evol. 17, 1–3. doi: 10.1016/S0169-5347(01)02381-3
Breck, S. W.. (2004). “Minimimzing carnivore-livestock conflict: the importance and process of research in the search for coexistence,” in People and Predators: From Conflict to Coexistence, eds N. Fascione, A. Delach, and M. Smith, 13–27.
Broekhuis, F., Cushman, S. A., Elliot, N. B., Cheetah, M., Trust, K. W., and Nairobi, K. (2017). Identification of human-carnivore conflict hotspots to prioritize mitigation efforts. Ecol. Evol. 7, 10630–10639. doi: 10.1002/ece3.3565
Chaka, S. N. M., Kissui, B. M., Gray, S., and Montgomery, R. A. (2020). Predicting the fine-scale factors that correlate with multiple carnivore depredation of livestock in their enclosures. Afr. J. Ecol. 59, 12789. doi: 10.1111/aje.12789
Chapin, F. S. I., Zavaleta, E. S., Eviner, V. T., Naylor, R. L., Vitousek, P. M., Reynolds, H. L., et al. (2000). Consequences of changing biodiversity. Nature 405, 234–242. doi: 10.1038/35012241
Cozzi, G., Broekhuis, F., Mcnutt, J. W., Turnbull, L. A., Macdonald, D. W., and Schmid, B. (2012). Fear of the dark or dinner by moonlight? Reduced temporal partitioning among Africa's large carnivores. Ecology 93, 2590–2599. doi: 10.1890/12-0017.1
Creel, S., and Christianson, D. (2008). Relationships between direct predation and risk effects. Trends Ecol. Evol. 23, 194–201. doi: 10.1016/j.tree.2007.12.004
Creel, S., Christianson, D., Liley, S., and Winnie, J. A. (2007). Predation risk affects reproductive physiology and demography of elk. Science 315, 960. doi: 10.1126/science.1135918
Curio, E.. (1976). The Ethology of Predation, ed D. S. Farner. Berlin; New York, NY: Springer-Verlag. doi: 10.1007/978-3-642-81028-2
Di Marco, M., Chapman, S., Althor, G., Kearney, S., and Watson, J. E. M. (2017). Changing trends and persisting biases in three decades of conservation science. Glob. Ecol. Conserv. 10, 32–42. doi: 10.1016/j.gecco.2017.01.008
Dickman, A. J.. (2010). Complexities of conflict: the importance of considering social factors for effectively resolving human-wildlife conflict. Anim. Conserv. 13, 458–466. doi: 10.1111/j.1469-1795.2010.00368.x
Dorresteijn, I., Schultner, J., Nimmo, D. G., Fischer, J., Hanspach, J., Kuemmerle, T., et al. (2015). Incorporating anthropogenic effects into trophic ecology: predator-prey interactions in a human-dominated landscape. Proc. R. Soc. B 282, 1602. doi: 10.1098/rspb.2015.1602
Eklund, A., López-Bao, J. V., Tourani, M., Chapron, G., and Frank, J. (2017). Limited evidence on the effectiveness of interventions to reduce livestock predation by large carnivores. Sci. Rep. 7, 209. doi: 10.1038/s41598-017-02323-w
Elliot, J. P., Cowan, I. M., and Holling, C. S. (1977). Prey capture by the African lion. Canad. J. Zool. 55, 1811–1828. doi: 10.1139/z77-235
Estes, J. A., Terborgh, J., Brashares, J. S., Power, M. E., Berger, J., Bond, W. J., et al. (2011). Trophic downgrading of planet earth. Science 333, 301–306. doi: 10.1126/science.1205106
Fortin, D., Boyce, M. S., Merrill, E. H., and Fryxell, J. M. (2004). Foraging costs of vigilance in large mammalian herbivores. Oikos 107, 172–180. doi: 10.1111/j.0030-1299.2004.12976.x
Frank, L.. (2010). “Living with lions: lessons from Laikipia,” in Conserving Wildlife in African Landscapes: Kenya's Ewaso Ecosystem, ed N. J. Georgiadis (Washington, DC: Smithsonian Institution Scholarly Press),73–83.
Frank, L., Hemson, G., Kushnir, H., Packer, C., and Maclennan, S. (2006). “Lions, conflict, and conservation,” in Management and Conservation of Large Carnivores in West and Central Africa: Proceedings of an International Seminar on the Conservation of Small and Hidden Species, eds B. Croes, R. Buij, H. de Iongh, and H. Bauer, 81–98.
Frank, L., Woodroffe, R., and Ogada, M. O. (2005). “People and predators in Laikipia District, Kenya,” in People and Wildlife: Conflict or Coexistence?, eds R. Woodroffe, S. Thirgood, and A. Rabinowitz (Cambridge: Cambridge University Press), 286–304. doi: 10.1017/CBO9780511614774.019
Frank, L. G.. (1998). Living with lions: Carnivore conservation and livestock in Laikipia District, Kenya. Available online at: http://www.lionconservation.org/ScientificPapers/Living-with-lions,Frank.pdf
Georgiadis, N. J., Olwero, J. G. N., Ojwang, G., and Romañach, S. S. (2007). Savanna herbivore dynamics in a livestock-dominated landscape: I. Dependence on land use, rainfall, density, and time. Biol. Conserv. 137, 461–472. doi: 10.1016/j.biocon.2007.03.005
Gray, S. M., Booher, C. R., Elliott, K. C., Kramer, D. B., Waller, J. C., Millspaugh, J. J., et al. (2019). Research-implementation gap limits the actionability of human-carnivore conflict studies in East Africa. Anim. Conserv. 23, 7–17. doi: 10.1111/acv.12520
Gray, T. N. E.. (2012). Studying large mammals with imperfect detection: status and habitat preferences of wild cattle and large carnivores in Eastern Cambodia. Biotropica 44, 531–536. doi: 10.1111/j.1744-7429.2011.00846.x
Hayward, M. W., Henschel, P., O 'brien, J., Hofmeyr, M., Balme, G., Kerley, G. I. H., et al. (2006). Prey preferences of the leopard (Panthera pardus). J. Zool. 270, 298–313. doi: 10.1111/j.1469-7998.2006.00139.x
Hebblewhite, M., Merrill, E. H., and McDonald, T. L. (2005). Spatial decomposition of predation risk using resource selection functions: an example in a wolf-elk predator-prey system. Oikos 111, 101–111. doi: 10.1111/j.0030-1299.2005.13858.x
Hoffmann, C. F., Kissui, B. M., and Montgomery, R. A. (2019). Spatial pattern analysis reveals randomness among carnivore depredation of livestock. Front. Ecol. Evol. 7, 1–10. doi: 10.3389/fevo.2019.00478
Hoffmann, C. F., and Montgomery, R. A. (2022). Implications of taxonomic bias for human-carnivore conflict mitigation. Oryx. 1–10. doi: 10.1017/S0030605321000582
Holling, C. S.. (1959). The components of predation as revealed by a study of small-mammal predation of the European pine sawfly. Canad. Entomol. 91, 293–320. doi: 10.4039/Ent91293-5
Hopcraft, J. G. C., Sinclair, A. R. E., Packer, C., and Packert, C. (2005). Planning for success: Serengeti lions seek prey accessibility rather than abundance. J. Anim. Ecol. 74, 559–566. doi: 10.1111/j.1365-2656.2005.00955.x
Kinnaird, M. F., and O'Brien, T. G. (2012). Effects of private-land use, livestock management, and human tolerance on diversity, distribution, and abundance of large African mammals. Conserv. Biol. 26, 1026–1039. doi: 10.1111/j.1523-1739.2012.01942.x
Kissui, B., Kiffner, C., Konig, H., and Montgomery, R. (2019). Patterns of livestock depredation and cost-effectiveness of fortified livestock enclosures in Northern Tanzania. Ecol. Evol. 9, 11420–11433. doi: 10.1002/ece3.5644
Kissui, B. M.. (2008). Livestock predation by lions, leopards, spotted hyenas, and their vulnerability to retaliatory killing in the Maasai steppe, Tanzania. Anim. Conserv. 11, 422–432. doi: 10.1111/j.1469-1795.2008.00199.x
Knight, A. T., Cowling, R. M., Rouget, M., Balmford, A., Lombard, A. T., and Campbell, B. M. (2008). Knowing but not doing: selecting priority conservation areas and the research-implementation gap. Conserv. Biol. 22, 610–617. doi: 10.1111/j.1523-1739.2008.00914.x
Kolowski, J. M., and Holekamp, K. E. (2006). Spatial, temporal, and physical characteristics of livestock depredations by large carnivores along a Kenyan reserve border. Biol. Conserv. 128, 529–541. doi: 10.1016/j.biocon.2005.10.021
Kolowski, J. M., and Holekamp, K. E. (2007). Effects of an open refuse pit on space use patterns of spotted hyenas. Afr. J. Ecol. 46, 341–349. doi: 10.1111/j.1365-2028.2007.00846.x
Krafte Holland, K., Larson, L. R., and Powell, R. B. (2018). Characterizing conflict between humans and big cats Panthera spp.: a systematic review of research trends and management opportunities. PLoS ONE 13, e0203877. doi: 10.1371/journal.pone.0203877
Kruuk, H.. (1972). The Spotted Hyena: A Study of Predation and Social Behavior. Chicago, IL: Echo Point Books and Media.
Kuiper, T. R., Loveridge, A. J., Parker, D. M., Johnson, P. J., Hunt, J. E., Stapelkamp, B., et al. (2015). Seasonal herding practices influence predation on domestic stock by African lions along a protected area boundary. Biol. Conserv. 191, 546–554. doi: 10.1016/j.biocon.2015.08.012
Laporte, I., Muhly, T. B., Pitt, J. A., Alexander, M., and Musiani, M. (2010). Effects of wolves on elk and cattle behaviors: implications for livestock production and wolf conservation. PLoS ONE 5, e11954. doi: 10.1371/journal.pone.0011954
Lawler, J. J., Aukema, J. E., Grant, J. B., Halpern, B. S., Kareiva, P., Nelson, C. R., et al. (2006). Conservation science: a 20-year report card. Front. Ecol. Environ. 4, 473–480. doi: 10.1890/1540-9295(2006)4[473:CSAYRC]2.0.CO;2
Leflore, E. G., Fuller, T. K., Tomeletso, M., and Stein, A. B. (2019). Livestock depredation by large carnivores in northern Botswana. Glob. Ecol. Conserv. 18, e00592. doi: 10.1016/j.gecco.2019.e00592
Lepard, C. C., Moll, R. J., Cepek, J. D., Lorch, P. D., Dennis, P. M., Robison, T., et al. (2019). The influence of the delay-period setting on camera-trap data storage, wildlife detections and occupancy models. Wildlife Res. 46, 37–53. doi: 10.1071/WR17181
Lichtenfeld, L. L., Trout, C., and Kisimir, E. L. (2015). Evidence-based conservation: predator-proof bomas protect livestock and lions. Biodiv. Conserv. 24, 483–491. doi: 10.1007/s10531-014-0828-x
Lima, S. L.. (2002). Putting predators back into behavioral predator -prey interactions. Trends Ecol. Evol. 17, 70–75. doi: 10.1016/S0169-5347(01)02393-X
Lima, S. L. (1998). Stress and decision making under the risk of predation: recent developments from behavioral, reproductive, and ecological perspectives. Adv. Study Behav/ 27, 215–290. doi: 10.1016/S0065-3454(08)60366-6
Lima, S. L., and Dill, L. M. (1990). Behavioral decisions made under the risk of predation: a review and prospectus. Canad. J. Zool. 68, 619–640. doi: 10.1139/z90-092
Linklater, W. L.. (2003). Science and management in a conservation crisis: a case study with rhinoceros. Conserv. Biol. 17, 968–975. doi: 10.1046/j.1523-1739.2003.01449.x
Loveridge, A. J., Kuiper, T., Parry, R. H., Sibanda, L., Hunt, J. H., Stapelkamp, B., et al. (2017). Bells, bomas and beefsteak: complex patterns of human-predator conflict at the wildlife-agropastoral interface in Zimbabwe. PeerJ. 5, e2898. doi: 10.7717/peerj.2898
MacNulty, D. R., Mech, L. D., and Smith, D. W. (2007). A proposed ethogram of large-carnivore predatory behavior, exemplified by the wolf. J. Mammal. 88, 595–605. doi: 10.1644/06-MAMM-A-119R1.1
Martín-López, B., Montes, C., Ramírez, L., and Benayas, J. (2009). What drives policy decision-making related to species conservation? Biol. Conserv. 142, 1370–1380. doi: 10.1016/j.biocon.2009.01.030
Mech, L. D.. (1970). “Hunting habits,” in The Wolf: The Ecology and Behavior of an Endangered Species (Garden City, NY: Natural History Press).
Miller, J. R., Jhala, Y. V., Jena, J., and Schmitz, O. J. (2015). Landscape-scale accessibility of livestock to tigers: implications of spatial grain for modeling predation risk to mitigate human-carnivore conflict. Ecol. Evol. 5, 1354–1367. doi: 10.1002/ece3.1440
Miller, J. R. B.. (2015). Mapping attack hotspots to mitigate human-carnivore conflict: approaches and applications of spatial predation risk modeling. Biodiv. Conserv. 24, 2887–2991. doi: 10.1007/s10531-015-0993-6
Miller, J. R. B., Stoner, K. J., Cejtin, M. R., Meyer, T. K., Middleton, A. D., and Schmitz, O. J. (2016). Effectiveness of contemporary techniques for reducing livestock depredations by large carnivores. Wildlife Society Bull. 40, 806–815. doi: 10.1002/wsb.720
Mizutani, F.. (1999a). Biomass density of wild and domestic herbivores and carrying capacity on a working ranch in Laikipia District, Kenya. Afr. J. Ecol. 37, 226–240. doi: 10.1046/j.1365-2028.1999.00171.x
Mizutani, F.. (1999b). Impact of leopards on a working ranch in Laikipia, Kenya. Afr. J. Ecol. 37, 211–225. doi: 10.1111/aje.1999.37.2.211
Moll, R. J., Redilla, K. M., Mudumba, T., Muneza, A. B., Gray, S. M., Abade, L., et al. (2017). The many faces of fear: a synthesis of the methodological variation in characterizing predation risk. J. Animal Ecol. 86, 749–765. doi: 10.1111/1365-2656.12680
Montgomery, R. A., Elliot, K. C., Hayward, M. W., Gray, S. M., Millspaugh, J. J., Riley, S. J., et al. (2018a). Examining evident interdisciplinarity among prides of lion researchers. Front. Ecol. Evol. 6, 49. doi: 10.3389/fevo.2018.00049
Montgomery, R. A., Hoffmann, C. F., Tans, E. D., and Kissui, B. (2018b). Discordant scales and the potential pitfalls for human-carnivore conflict mitigation. Biol. Conserv. 224, 170–177. doi: 10.1016/j.biocon.2018.05.018
Mpakairi, K., Ndaimani, H., Vingi, K., Madiri, T. H., and Nekatambe, T. (2018). Ensemble modelling predicts human carnivore conflict for a community adjacent to a protected area in Zimbabwe. Afr. J. Ecol. 56, 957–963. doi: 10.1111/aje.12526
Mponzi, P., Lepczyk, C. A., and Kissui, B. M. (2014). Characteristics and distribution of livestock losses caused by wild carnivores in Maasai Steppe of northern Tanzania. Human–Wildlife Interactions 8, 218–227. doi: 10.26077/ydcm-0b38
Mukeka, J. M., Ogutu, J. O., Kanga, E., and Røskaft, E. (2019). Human-wildlife conflicts and their correlates in Narok County, Kenya. Glob. Ecol. Conserv. 18, e00620. doi: 10.1016/j.gecco.2019.e00620
Ogada, M. O., Woodroffe, R., Oguge, N. O., and Frank, L. G. (2003). Limiting depredation by African carnivores: the role of livestock husbandry. Conserv. Biol. 17, 1521–1530. doi: 10.1111/j.1523-1739.2003.00061.x
Patterson, B. D., Kasiki, S. M., Selempo, E., and Kays, R. W. (2004). Livestock predation by lions (Panthera leo) and other carnivores on ranches neighboring Tsavo National Park, Kenya. Biol. Conserv. 119, 507–516. doi: 10.1016/j.biocon.2004.01.013
Pimm, S. L., Jenkins, C. N., Abell, R., Brooks, T. M., Gittleman, J. L., Joppa, L. N., et al. (2014). The biodiversity of species and their rates of extinction, distribution, and protection. Science 344, 1246752. doi: 10.1126/science.1246752
Pozo, R. A., Leflore, E. G., Duthie, A. B., Bunnefeld, N., Jones, I. L., Minderman, J., et al. (2020). A multispecies assessment of wildlife impacts on local community livelihoods. Conserv. Biol. 35, 297–306. doi: 10.1111/cobi.13565
Prager, K. C., Mazet, J. A. K., Dubovi, E. J., Frank, L. G., Munson, L., Wagner, A. P., et al. (2012). Rabies virus and canine distemper virus in wild and domestic carnivores in Northern Kenya: are domestic dogs the reservoir? EcoHealth 9, 483–498. doi: 10.1007/s10393-013-0815-9
Prugh, L. R., Sivy, K. J., Mahoney, P. J., Ganz, T. R., Ditmer, M. A., van de Kerk, M., et al. (2019). Designing studies of predation risk for improved inference in carnivore-ungulate systems. Biol. Conserv. 232, 194–207. doi: 10.1016/j.biocon.2019.02.011
Ripple, W. J., Chapron, G., López-Bao, J. V., Durant, S. M., Macdonald, D. W., Lindsey, P. A., et al. (2016). Saving the world's terrestrial Megafauna. BioScience 66, 807–812. doi: 10.1093/biosci/biw092
Ripple, W. J., Estes, J. A., Beschta, R. L., Wilmers, C. C., Ritchie, E. G., Hebblewhite, M., et al. (2014). Status and ecological effects of the world's largest carnivores. Science 343, 151–162. doi: 10.1126/science.1241484
Robertson, J. A. D., Roodbol, M., Bowles, M. D., Dures, S. G., and Rowcliffe, J. M. (2019). Environmental predictors of livestock predation: a lion's tale. Oryx 54, 1–10. doi: 10.1017/S0030605318001217
Ruppert, K. A., Laiyon, L., Letoluai, A., Owen, M. A., Pilfold, N. W., Wachira, P., et al. (2021). Avoiding parachute science when addressing conflict over wildlife. Conserv. Sci. Pract. e548. doi: 10.1111/csp2.548
Stroud, J. T., Rehm, E., Ladd, M., Olivas, P., and Feeley, K. J. (2014). Is conservation research money being spent wisely? Changing trends in conservation research priorities. J. Nat. Conserv. 22, 471–473. doi: 10.1016/j.jnc.2014.05.003
Sunderland, T., Sunderland-Groves, J., Shanley, P., and Campbell, B. (2009). Bridging the gap: How can information access and exchange between conservation biologists and field practitioners be improved for better conservation outcomes? Biotropica 41, 549–554. doi: 10.1111/j.1744-7429.2009.00557.x
Treves, A., Naughton-Treves, L., Harper, E. K., Mladenoff, D. J., Rose, R. A., Sickley, T. A., et al. (2004). Predicting human-carnivore conflict: a spatial model derived from 25 years of data on wolf predation on livestock. Conserv. Biol. 18, 114–125. doi: 10.1111/j.1523-1739.2004.00189.x
Troudet, J., Grandcolas, P., Blin, A., Vignes-Lebbe, R., and Legendre, F. (2017). Taxonomic bias in biodiversity data and societal preferences. Nat. Sci. Rep. 7, 9132. doi: 10.1038/s41598-017-09084-6
Ulrich, A., Ifejika Speranza, C., Roden, P., Kiteme, B., Wiesmann, U., and Nüsser, M. (2012). Small-scale farming in semi-arid areas: Livelihood dynamics between 1997 and 2010 in Laikipia, Kenya. J. Rural Stud. 28, 241–251. doi: 10.1016/j.jrurstud.2012.02.003
Unks, R. R., King, E. G., German, L. A., Wachira, N. P., and Nelson, D. R. (2019). Unevenness in scale mismatches: Institutional change, pastoralist livelihoods, and herding ecology in Laikipia, Kenya. Geoforum 99, 74–87. doi: 10.1016/j.geoforum.2018.12.010
Valeix, M., Hemson, G., Loveridge, A. J., Mills, G., and Macdonald, D. W. (2012). Behavioural adjustments of a large carnivore to access secondary prey in a human-dominated landscape. J. Appl. Ecol. 49, 73–81. doi: 10.1111/j.1365-2664.2011.02099.x
Valeix, M., Loveridge, A. J., Chamaillé-Jammes, S., Davidson, Z., Murindagomo, F., Fritz, H., et al. (2009). Behavioral adjustments of African herbivores to predation risk by lions: spatiotemporal variations influence habitat use. Ecology 90, 23–30. doi: 10.1890/08-0606.1
Van Cleave, E. K., Bidner, L. R., Ford, A. T., Caillaud, D., Wilmers, C. C., and Isbell, L. A. (2018). Diel patterns of movement activity and habitat use by leopards (Panthera pardus pardus) living in a human-dominated landscape in central Kenya. Biol. Conserv. 226, 224–237. doi: 10.1016/j.biocon.2018.08.003
Van der Weyde, L. K., Mbisana, C., and Klein, R. (2018). Multi-species occupancy modelling of a carnivore guild in wildlife management areas in the Kalahari. Biol. Conserv. 220, 21–28. doi: 10.1016/j.biocon.2018.01.033
van Eeden, L. M., Crowther, M. S., Dickman, C. R., Macdonald, D. W., Ripple, W. J., Ritchie, E. G., et al. (2018a). Managing conflict between large carnivores and livestock. Conserv. Biol. 32, 26–34. doi: 10.1111/cobi.12959
van Eeden, L. M., Eklund, A., Miller, J. R. B., López-Bao, V., Chapron, G., Cejtin, M. R., et al. (2018b). Carnivore conservation needs evidence-based livestock protection. PLoS Biol. 16, e2005577. doi: 10.1371/journal.pbio.2005577
Wagner, A. P.. (2006). Behavioral Ecology of the Striped Hyena (Hyaena hyaena). Bozeman, MT: Montana State University.
Wilmers, C. C., Nickel, B., Bryce, C. M., Smith, J. A., Wheat, R. E., and Yovovich, V. (2015). The golden age of bio-logging: how animal-borne sensors are advancing the frontiers of ecology. Ecology 96, 1741–1753. doi: 10.1890/14-1401.1
Wolf, C., and Ripple, W. J. (2017). Range contractions of the world's large carnivores. R. Soc. Open Sci. 4, 170052. doi: 10.1098/rsos.170052
Woodroffe, R., and Frank, L. G. (2005). Lethal control of African lions (Panthera leo): local and regional population impacts. Anim. Conserv. 8, 91–98. doi: 10.1017/S1367943004001829
Woodroffe, R., Lindsey, P., Romañach, S., Stein, A., and Ole Ranah, S. M. K. (2005). Livestock predation by endangered African wild dogs (Lycaon pictus) in northern Kenya. Biol. Conserv. 124, 225–234. doi: 10.1016/j.biocon.2005.01.028
Yirga, G., De Iongh, H. H., Leirs, H., Gebrehiwot, K., Berhe, G., Asmelash, T., et al. (2012). The ecology of large carnivores in the highlands of northern Ethiopia. Afr. J. Ecol. 51, 78–86. doi: 10.1111/aje.12008
Yirga, G., De Iongh, H. H., Leirs, H., Kiros, S., Yohannes, T. G., Teferi, M., et al. (2014). Local spotted hyena abundance and community tolerance of depredation in human-dominated landscapes in Northern Ethiopia. Mammal. Biol. 79, 325–330. doi: 10.1016/j.mambio.2014.05.002
Yirga, G., Leirs, H., De Iongh, H. H., Asmelash, T., Gebrehiwot, K., Deckers, J., et al. (2015). Spotted hyena (Crocuta crocuta) concentrate around urban waste dumps across Tigray, northern Ethiopia. Wildlife Res. 42, 563. doi: 10.1071/WR14228
Keywords: conflict management, human-carnivore conflict, livestock depredation, predation risk, motion-activated camera
Citation: Hoffmann CF, Pilfold NW, Ruppert KA, Letoluai A, Lenguya L, Limo I and Montgomery RA (2022) The Integral Nature of Encounter Rate in Predicting Livestock Depredation Risk. Front. Conserv. Sci. 3:808043. doi: 10.3389/fcosc.2022.808043
Received: 02 November 2021; Accepted: 07 February 2022;
Published: 09 March 2022.
Edited by:
Adam T. Ford, University of British Columbia, CanadaReviewed by:
Ali T. Qashqaei, Independent Researcher, Tehran, IranKatherine Whitehouse-Tedd, Nottingham Trent University, United Kingdom
Copyright © 2022 Hoffmann, Pilfold, Ruppert, Letoluai, Lenguya, Limo and Montgomery. This is an open-access article distributed under the terms of the Creative Commons Attribution License (CC BY). The use, distribution or reproduction in other forums is permitted, provided the original author(s) and the copyright owner(s) are credited and that the original publication in this journal is cited, in accordance with accepted academic practice. No use, distribution or reproduction is permitted which does not comply with these terms.
*Correspondence: Claire F. Hoffmann, clairefhoffmann@gmail.com